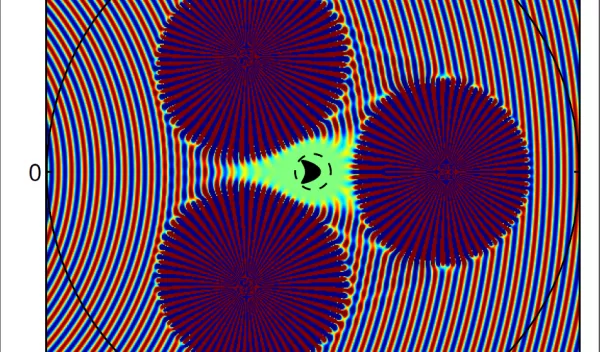
Hidden from view
The idea of cloaking and rendering something invisible hit the small screen in 1966 when a Romulan Bird of Prey made an unseen, surprise attack on the Starship Enterprise on Star Trek. Not only did it make for a good storyline, it likely inspired budding scientists, offering a window of technology's potential.
Today, between illusionists who make the Statue of Liberty disappear to Harry Potter's invisibility cloak that not only hides him from view but also protects him from spells, pop culture has embraced the idea of hiding behind force fields and magical materials. And not too surprisingly, National Science Foundation (NSF)-funded mathematicians, scientists and engineers are equally fascinated and looking at how and if they can transform science fiction into, well, just science.
"Cloaking is about detection and rendering something--and the cloak itself--not detectable or seen," said Michael Weinstein, an NSF-funded mathematician at Columbia University. "An object is seen when waves are bounced off it and observed by a detector."
In recent years, researchers have developed new ways in which light can move around and even through a physical object, making it invisible to parts of the electromagnetic spectrum and undetectable by sensors. Additionally, mathematicians, theoretical physicists and engineers are exploring how and whether it's feasible to cloak against other waves besides light waves. In fact, they are investigating sound waves, sea waves, seismic waves and electromagnetic waves including microwaves, infrared light, radio and television signals.
Successful outcomes have far-reaching results--like protecting deep-water oil rigs from earthquakes and vulnerable beaches from tsunamis.
Uncloaking cloaking's math and science history
Partial differential equations, coordinate invariance, wave equations--when you start talking to researchers about cloaking, it soon starts sounding a lot like math. And that's because at the very heart of this scientific question lies a mathematical one.
"There are very nice mathematical problems associated with this, and some of the ideas are mathematically very, very simple," said Michael Vogelius, NSF's division director for mathematical sciences and whose own research at Rutgers University has contributed significantly to this field. "But that doesn't mean they are simple to implement. In transformation cloaking the materials with the desired cloaking properties are found by singular or nearly singular change of variables in the energy expression--these material coefficients are sometimes referred to as the push-forward (or pull-back) of the original background. Basically, mathematicians ask, 'what do the equations have to look like to get this effect?' The thing that will be very hard--and is very hard--is to build these materials. They are singular in all kinds of ways."
That is why throughout cloaking research history, mathematicians, theoretical physicists and engineers have looked at the problem together.
According to Graeme Milton, an NSF-funded mathematician at the University of Utah, cloaking's start is rooted in math.
"Mathematicians and theoretical physicists basically had the idea independently for transformation-based cloaking," he said, adding that other mathematicians along the way--including himself--have taken the same wave equations and developed them further.
Milton and his collaborators created superlens cloaking, where cloaking occurs near lenses with capabilities far greater than traditional ones, and active exterior cloaking, where cloaking is created by active devices, and the cloak does not completely surround the object.
While cloaking has made considerable theoretical strides, its triumphs have been fairly limited for those awaiting real-world applications.
"Essentially, all the cloaking that has been done successfully in experiment involves a fixed frequency or small band of frequencies," Weinstein said. "So, it's a bit like--suppose you detect things by shining a light on them, and we all agree you're only allowed to shine blue light. I can construct a cloak that will conceal it under blue light, but if you vary the color--that is the wavelength--of the probing light, it will then be detectable. So far, we are unable to cloak something that is invisible to all colors. And because white light is composed of a broad spectrum of colors, no one has come near to making things truly undetectable."
Even with those limitations, there have been distinct milestones in cloaking research.
One of the best examples is actually widely available but probably not commonly thought of as cloaking technology, yet it applies the same sort of math. It involves sounds waves.
"Noise-cancelling headphones are basically cloaking the sounds from outside so they don't reach your ears," Milton said. "Active cloaking is very much along these same lines."
In 2006, as Milton published a key paper that expanded on the superlens cloaking he developed more than a decade earlier, a group of Duke University physicists created the first-ever microwave invisibility cloak using specially engineered "metamaterials," which can manipulate wavelengths, such as light, in a way that naturally occurring materials cannot do alone. However, it only cloaked microwaves and only in two dimensions.
And in 2014, a group in France actually did some experiments with a company to drill 5-meter-deep holes in strategic locations that would modify the earth's density and then measure effectiveness in cloaking. The experiments man-made vibrations that were at a given frequency, not earthquakes. They were able to deflect the seismic waves, showing some possibility to develop this application further.
"Science needs to figure out how to cloak against multiple frequencies before there can be any 'real' cloaking, however," Milton said. "Earthquakes and tsunamis involve a mixture of frequencies, so they are particularly challenging problems."
Passive and active approaches to cloaking research
To understand cloaking, one must first understand where the idea comes from.
When light encounters an object, it is either reflected, refracted or absorbed. Reflection means light waves bounce off an object, like a mirror. Refraction bends light waves, much like looking at a straw in a glass of water seems to break the straw into two pieces. When waves are absorbed, they are stopped, neither bouncing back nor transmitting through the object--although perhaps heating it. Objects which absorb light appear opaque or dark. These interactions between light and objects are what allow us to see those objects.
For cloaking to occur, light must be tricked into doing unusual things that reduce our ability to "see" or detect the object. Mathematicians look for how to control the flow of waves, using wave equations to characterize their behavior. Wave equations are an example of partial differential equation (PDE); PDEs are the language of the fundamental laws of physics. (Just this year, John Nash and Louis Nirenberg received the prestigious Abel Prize for their work in partial differential equations. Their contributions have had a major impact on how mathematicians analyze the PDEs used to understand phenomena such as cloaking.)
"All wave phenomena are predictable from these wave equations--at least in principle," Weinstein said. "That is, light waves, sound waves, elastic waves, quantum waves, gravitational waves. But the problem is that these equations are not so easily solved, so one tries to come up with guiding principles, useful approximations and rules of thumb. Coming to the question of cloaking, there's a mathematical property of wave equations, governing, for example, light, called coordinate invariance. That's basically a way of saying that you can change coordinates and perspectives of viewing the object, and the equations themselves don't change their essential form. By exploiting this idea of coordinate invariance, scientists have come up with prescriptions for optical properties that can cloak arbitrary objects."
In 2009, Milton and colleagues first introduced exterior active cloaking. Scientists in this field describe their research as involving either active or passive cloaking. Active cloaking uses devices that actively generate electromagnetic fields that distort waves. Passive cloaking employs metamaterials that passively shield objects from electromagnetic waves rather than intervening.
"The term 'metamaterial' is a bit deceptive," Weinstein noted. "Metamaterials are roughly composite materials. You take a bunch of building blocks, made from naturally occurring materials, and put them together in interesting ways to create some emergent property--some collective property of this novel arrangement not in naturally occurring materials. That new collective material is a metamaterial. But it's more like a device that actually interacts actively with waves moving through it."
With new metamaterial designs come new cloaking capabilities. NSF-funded engineer Andrea Alù won NSF's Waterman award in 2015 for creating metamaterials that can cloak a three-dimensional object. He and his team developed two methods--plasmonic cloaking and mantle cloaking--that take advantage of different light-scattering effects to hide an object.
Weinstein is exploring, through his research on the partial differential equations governing light, electromagnetism, sound, etc.--different ways of controlling the flow of energy, cloaking being one example, by using novel media such as metamaterials. Vogelius is known for bringing credibility to the transformation optics that serve as a backbone to cloaking broadly.
Where's my invisibility cloak?
But most fans of stealthful space ships, submarines and cloaks will still wonder: how close are we to really having any of this technology?
"I think that from the perspective of lay people, the most misunderstood thing is thinking this technology is right around the corner," Milton said. "Realistic Harry Potter cloaks are still a long way off."
Unfortunately, addressing multi-frequency cloaking will take time.
"What I do see is a merging of mathematical, physical and engineering principles to more effectively enable isolation of objects from harmful environments--there will be movement in that direction," Weinstein said. "Also, there will be important experimental advances resulting from attempts to achieve what is only theoretically possible at this time."
In the meantime, these mathematicians often look at other issues--sometimes similar ones that offer the potential to rethink their approaches.
"Right now, we're working on the opposite sort of problem--on the limitations to cloaking," Milton said. "Cloaking is just one of the many avenues I work on. Honestly, it's always stimulating to explore the limitations of what's possible and what's impossible."