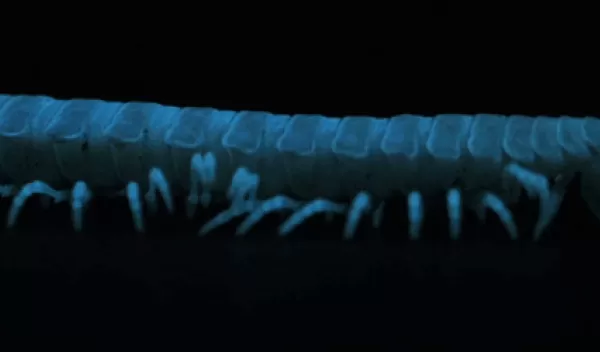
Night lights: The wonders of bioluminescent millipedes
Editor's Note: This Behind the Scenes article was first provided to LiveScience in partnership with the National Science Foundation.
There's something inherently magical, even surreal, about seeing hundreds of glowing millipedes scattered across the ground of a sequoia grove on a moonless night in Sequoia National Park.
Every evening, these creatures--which remain hidden underground during the day--emerge and initiate a chemical reaction to produce a green-blue glow, a process called bioluminescence. The eerie night lights of these millipedes highlight nature’s eccentricities. My observations of this phenomena is a fringe benefit of my research of the millipede species known as Motyxia.
Seeing the light
Motyxia, which are the only known bioluminescent millipedes, are found solely in a small region of the Sierra Nevada mountain range in California. But various types of bioluminescent creatures live throughout the United States. They include:
- railroad worms, a beetle that looks similar to a millipede but has a string of lights down each of its sides resembling the lit windows of a passenger train at night,
- glowworms with bioluminescent lamps on their heads,
- a fly larvae with the bluest bioluminescence in the insect world,
- firefly larvae that have two abdominal lamps on their tail,
- and even luminescent earthworms.
If you would like to see bioluminescent creatures, visit a moist area, such as a gully or streamside, in a deep dark forest late at night--preferably in the early summer, right after a rain.
When you arrive at your viewing sight, turn off your flashlight and let your eyes adjust to the dark. Within about 15 to 30 minutes, you may begin to discern bioluminescent organisms.
Focus on tiny specks of light, which may be firefly larvae. These organisms may quickly turn off their lights when approached--but then turn them on again. So if you initially see a twinkle, note its position relative to nearby stationary objects so that you may see it light up again.
If you want to light your path as you walk, use red light to maintain your light-adapted vision.
Why the turn on?
When you observe bioluminescence, you may wonder about the purpose of this illuminating phenomenon. My research on Motyxia indicates that "Glow means 'No!'" to predators. That is, Motyxia's glow warns nocturnal predators that these 60-legged creatures are armed and dangerous; any predator that riles a Motyxia risks being squirted by toxins, including hydrogen cyanide, an extremely poisonous gas, which the millipede releases when it feels threatened.
The suggestion that Motyxia's glow wards off marauding nocturnal predators is supported by the fact that Motyxia are blind, so their visual signaling can only be seen by members of other species, such as predators.
My research team and I ran an experiment to test whether Motyxia's coloration warns predators to stay away. Our experiment involved positioning 150 glowing clay millipede models and 150 clay non-glowing millipede models in Motyxia's natural nighttime habitat in California.
The results: Predators attacked a significantly lower percentage of the glowing vs. non-glowing models (18 percent vs. 49 percent.) The relatively greater ability of the glowing millipede models to repel predators supports the "Glow Means No!" idea.
Motyxia's eastern cousins possess bright and conspicuous reds and yellows, apparently also to ward off daytime predators.
Other animals that are toxic, inedible, or otherwise noxious also advertise their danger via warning signals. For example, a rattlesnake uses its rattle and the yellow jacket brandishes yellow and black stripes to advertise its threats.
Toxic animals that show bright, highly conspicuous and sometimes downright garish colors to distinguish themselves thereby help prevent predators from mistaking them for edible prey. Such an error would be costly to both predator and prey.
The conspicuous appearance of toxic animals also helps predators learn to recognize their bright coloration as warnings and remember the unpleasant consequences of ignoring them--e.g. a cyanide-induced fever.
How bioluminescence evolved
How did bioluminescence evolve? This question is another focus of our ongoing research on Motyxia.
By helping to reveal the evolutionary origins of warning colorations--which, by necessity, contribute to some of the most blatant and complex appearances in the living world--we expect to improve our ability to investigate and understand how other complex traits arise in nature.
One possible clue to the origins of bioluminescence is provided by a millipede species known as Motyxia sequoiae, which inhabits habitats that are normally off-limits to other closely related millipedes. These habitats include exposed areas of the forest floor, open mountain meadows and the trunks of oak trees.
So perhaps bioluminescence evolved in Motyxia sequoiae to protect these creatures from predators in particularly vulnerable areas, and thereby enable these millipedes to expand their range to these favorable locations.
But why would Motyxia sequoiae evolve bioluminescence instead of any other defense mechanism, such as camouflage or weapons such as claws or sharp spines?
Have you ever heard the saying that "natural selection...works like a tinkerer"? This is a great way to think about the evolution of warning coloration and other complex biologic features. Tinkerers use what's already available (e.g., odds and ends lying around) to repair machines, appliances and other apparatuses.
A body of research suggests that many species may have similarly acquired bioluminescence by "making do" with, or repurposing, biological equipment they already possessed.
For example, fireflies need an enzyme called luciferase to light up. But the original role of the firefly's luciferase wasn't to help these insects produce light, but instead to help them synthesize fatty acids needed to create brain cells.
The essence of bioluminescence
Despite our growing knowledge, much about Motyxia remains mysterious. For example, how do these blind creatures find mates? What triggers their nightly emergence? With funding from the National Science Foundation, my team is working to answer these and other questions.
This research is part of our larger effort to describe biodiversity and reconstruct the evolutionary histories of arthropods--a group that includes insects, spiders and crustaceans, and accounts for 80 percent of all living species. We contribute our findings to the Tree of Life, which is a worldwide effort to define the evolutionary histories of animals.
Some bright ideas from bioluminescence
In addition to advancing our understanding of the history of life, studies of the bioluminescence of various types of organisms have implications for fields ranging from national defense to medicine.
Here are several examples:
- The efficiency of electrical lighting systems, which can be only 10 percent efficient, could be improved by designing them to mimic bioluminescent light, which is 90 percent efficient.
- The underbellies of some marine bioluminescent animals blend with background light from the water's surface, and so are camouflaged. The U.S. Navy is studying these phenomena so that it may build similarly camouflaged ships.
- Healthy human cells produce ultra-weak amounts of light through a process similar to animal bioluminescence, but cancer cells produce slightly more light. Techniques may ultimately be developed to help locate cancer cells by detecting the greater amounts of light they produce.
- A green fluorescent protein identified in a jellyfish species is now widely used in biomedical research as a fluorescent tag to help researchers track specific biological activities, such as the spread of cancer, insulin production and the movement of HIV proteins.
- The key enzyme for beetle bioluminescence is a pivotal component of a fast, inexpensive method for sequencing genomes, which in 2008 was used to sequence the full genome of a Neanderthal.
Learn more about Dr. Marek's work at jointedlegs.org