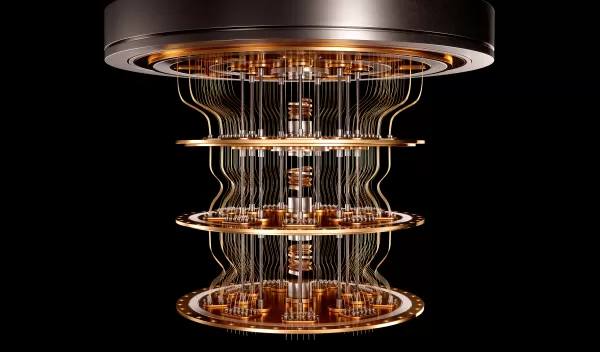
Self-correcting quantum computers within reach?
Quantum computers promise to reach speeds and efficiencies impossible for even the fastest supercomputers of today. But quantum computers, unlike classical ones, cannot correct errors by copying encoded data over and over. Because of this inability to self-correct, the technology hasn't seen much scale-up and commercialization.
Now, a paper in Nature illustrates a Harvard University quantum computing platform's potential to solve the long-standing problem known as quantum error correction. The work was a collaboration among researchers at Harvard, MIT, and Boston-based QuEra Computing. One of the paper's authors, Harvard's Dolev Bluvstein, was a recent U.S. National Science Foundation Graduate Research Fellow. The research is also underway through NSF’s Physics Frontiers Center for Ultracold Atoms.
An effort spanning the last several years, the Harvard platform is built on an array of very cold, laser-trapped rubidium atoms. Each atom acts as a bit, a "qubit," which can perform extremely fast calculations.
The team's chief innovation is configuring its "neutral atom array" to change its layout by moving and connecting atoms, called "entangling." Operations that entangle pairs of atoms, called two-qubit logic gates, are units of computing power.
Running a complicated algorithm on a quantum computer requires many gates. However, these gate operations are notoriously error-prone, and a buildup of errors renders the algorithm useless.
In the paper, the team reports near-flawless performance of its two-qubit entangling gates with extremely low error rates. For the first time, the researchers demonstrated the ability to entangle atoms with error rates below 0.5%. In terms of operation quality, that puts the technology's performance on par with other leading types of quantum computing platforms, like superconducting qubits and trapped-ion qubits.
However, the approach has major advantages over these competitors due to its large system sizes, efficient qubit control and ability to dynamically reconfigure the layout of atoms.