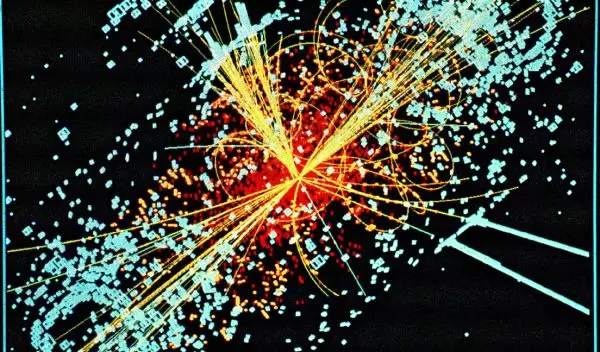
Testing Technicolor Physics
As the Large Hadron Collider (LHC) ramps up the rate and impact of its collisions, physicists hope to witness the emergence of the Higgs boson, an anticipated, but as-yet-unseen, fundamental particle that scientists believe gives mass to matter.
The Higgs boson is a central component of the "standard model," a theory that defines the relationships between the forces of the universe. But, what if the Higgs boson is not a fundamental particle, but rather a bound state of new particles that have not yet been seen?
"From the beginning of the standard model, people have been unhappy with the idea that the Higgs boson is a fundamental particle," said Thomas DeGrand, professor of physics at the University of Colorado.
Scientists who advocate alternative models of particle physics are motivated by the theory of superconductivity. The superconducting state, which is very different from ordinary matter, is not characterized by new particles, but by Cooper pairs--bound states of electrons.
The standard model of particle physics suggests that the protons and neutrons we're familiar with, that comprise the nuclei of atoms, are made up of combinations of different types of elementary particles called quarks and gluons. We cannot see these particles in isolation because a fundamental force called "the strong interaction" holds them firmly together, but experiments lead physicists to believe these quarks come in three varieties, each with different properties, or "colors".
No one knows why the particle content of the standard model is what it is. A logical possibility exists that there could be more kinds of quarks and gluons with different numbers of colors, strongly interacting with each other. Collectively, these possibilities are known as technicolor theories.
DeGrand spent many years studying the theory behind the interactions of quarks and gluons, known as quantum chromodynamics (QCD), before he turned his attention to technicolor theories. He is the co-author of one of the standard books in the field.
However, QCD does not describe all aspects of the standard model, especially the nature of "final" undiscovered particles. The construction of the LHC, and the sense that new knowledge was waiting around the corner, drew DeGrand to explorations of alternative particle theories.
"I became a little tired of lattice QCD," DeGrand said. "The technicolor theories held more interesting questions."
Within the last five years, scientists realized that many of the computational techniques that had been invented for QCD could be applied technicolor theories as well. In 2008, two colleagues from Tel Aviv University, Yigal Shamir and Benjamin Svetitsky, invited DeGrand to join their research team. Applying the same methodology DeGrand helped perfect for QCD, the team began simulating technicolor candidate theories and drawing conclusions from the odd outcomes of these simulated worlds.
To calculate the interactions of new kinds of quarks and gluons in various configurations on lattices of giant grids, the team used the National Science Foundation (NSF)-funded Ranger supercomputer--one of the largest in the world--located at the Texas Advanced Computing Center. In the numerical simulations, the grids are housed (virtually) in boxes of various sizes and the reaction of the particles to the box size provides information about the energy characteristics of the system.
"This is not something an experimentalist can do," DeGrand said. "But as theorists, we can invent these fake worlds where the system sits in a specific sized box, and we can measure the strength of the quark-gluon interactions in large boxes, medium boxes and small boxes to see how it changes. Changing the energy or momentum scales is related to changing the physical size of the system."
Supercomputing plays a pivotal role in this process, which involves solving complex quantum equations for a large number of particles. Over the last two years, DeGrand and his colleagues have used nearly three million processing hours on Ranger (the equivalent of 340 years on a single processor) to simulate new particles comprised of quarks with two colors and three colors, respectively. The simulations help characterize the new particles and determine whether they are candidates for beyond-the-standard-model physics.
The Ranger simulations revealed that the simplest technicolor models, with two colors, have properties that are very different from a conventional particle system. Professor H. Georgi of Harvard University coined the name "unparticle theory" to describe such systems.
The three-color system was a bit more mysterious. The researchers couldn't tell if it was a particle theory or an unparticle theory. However, the simulations clearly didn't represent a viable real-world scenario. For a technicolor theory to be a feasible candidate for new physics, it must exhibit unusual behavior to avoid conflict with the constraints defined by present-day experimental knowledge. The three-color system didn't satisfy these criteria. The researchers are currently continuing their map of possible particle models by simulating four-color quark systems.
"The idea that the Higgs mechanism could be caused by the strong interactions of still-to-be-discovered elementary particles has been with us for some time, but until recently, it has been difficult to test this idea for lack of adequate computing resources," said Carlton DeTar, a longtime collaborator from the University of Utah not involved in the current research. "DeGrand and his collaborators are among the foremost groups in the world using powerful numerical simulations to investigate this exciting alternative. The results could have profound implications for the search for the Higgs particle at the Large Hadron Collider in Europe."
Just as Salvador Dali's surreal paintings reveal taken-for-granted aspects of our material world, the alternative technicolor theories have intellectual value. They teach us about particle theories by placing them in a larger context.
For DeGrand, the stretching of the mind is what matters most--that, and the adventure of discovering something truly novel.
"It's high-risk, high-reward research," DeGrand said.
But, if he and his colleagues find a viable alternative to the standard model, it could drive the next theory of everything.
The work was also supported by the U.S. Department of Energy. Additional computations were done on facilities of the USQCD Collaboration at Fermilab, which are funded by the Office of Science of the U.S. Department of Energy.
-- Aaron Dubrow, Texas Advanced Computing Center, aarondubrow@tacc.utexas.edu
This Behind the Scenes article was provided to LiveScience in partnership with the National Science Foundation.