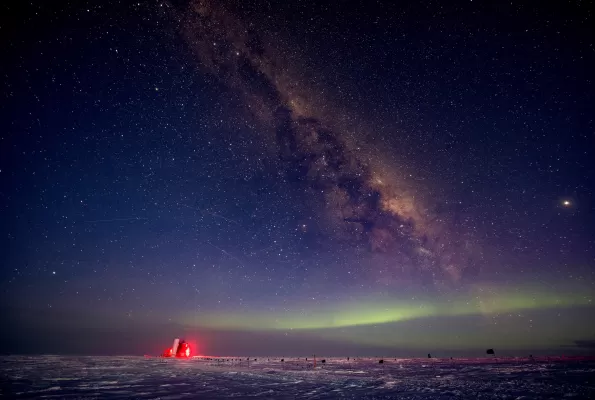
3 things learned from IceCube's first 10 years
The world's largest neutrino detector gives researchers a way to probe some of the most violent processes in the universe
Neutrinos are tiny, nearly massless elementary particles that rarely interact with normal matter. They were first made during the Big Bang and are continuously produced today by stars, black holes and other cosmic structures. Neutrinos are everywhere – billions pass through a square centimeter of Earth every second – but are difficult to detect and study.
The largest neutrino observatory in the world, the IceCube Neutrino Observatory, consists of thousands of sensors draped through a cubic kilometer of ice at the geographic South Pole. It was built to study cosmic neutrinos – those that come from outside the solar system and are made in powerful cosmic objects like black holes and pulsars.
Studying neutrinos is important for understanding the makeup of the universe, but IceCube, operated by the University of Wisconsin–Madison and supported by the U.S. National Science Foundation, was designed to use neutrinos as an astronomical messenger: to tell researchers about the violent, chaotic environments in which they were created.
In its first decade of operations, the ice-encased detector has given researchers new ways of looking at the cosmos. "Whenever we look at the universe with a new messenger, a particle we hadn't had the capability to exploit before, we always learn new things," said Dawn Williams, a physicist at the University of Alabama and member of the IceCube collaboration. The IceCube Observatory was "built to exploit this messenger – to use neutrinos to explore the universe, and we have succeeded ... beyond our wildest dreams."
Here are three things scientists have learned from IceCube's first decade of science and a peek at what physicists hope to learn in the future.
High-energy neutrinos are being made outside the solar system
One of the first things physicists learned from IceCube is that there is indeed a flux of high-energy cosmic neutrinos detectable on Earth. Before IceCube was built, physicists had observed cosmic neutrinos directly only once before, when light and particles from a supernova reached Earth in 1987. Observatories around the world picked up 25 neutrinos from the explosion of a star in the Large Magellanic Cloud, a small companion galaxy of the Milky Way. But those neutrinos were low in energy. High-energy neutrinos from cosmic accelerators like black holes are much rarer and harder to detect.
In 2013, IceCube scientists announced they had detected 28 high-energy neutrinos, which was the first solid evidence for neutrinos coming from cosmic accelerators outside the solar system. These neutrinos were a million times more energetic than those from the 1987 supernova.
Neutrino astronomy is a real thing
A few years after discovering a flux of cosmic neutrinos, IceCube accomplished its second major goal: identifying a candidate source of high-energy neutrinos. Physicists knew neutrinos are made in chaotic environments like black holes, but they had never pinpointed a specific object as being a high-energy neutrino "factory."
In 2017, IceCube scientists picked up a high-energy neutrino they traced to a flaring blazar, a giant elliptical galaxy with a supermassive black hole at its center. Black holes at the center of blazars have twin jets that spew light and elementary particles from their poles.
That high-energy neutrino triggered IceCube's automated alert system, which directed telescopes around the world to home in on the area of sky from which the neutrino originated. Several telescopes noticed a flare of gamma rays coming from a blazar about 4 billion light-years away. Astrophysicists concluded that this was the source of both the gamma rays and the high-energy neutrino they observed.
Physicists then looked at past IceCube observations and found a bigger flux of neutrinos from three years earlier that originated from the same area of the sky – and presumably from the same blazar.
This discovery was significant not only because it was the first time a high-energy neutrino source had been confirmed, but also because it ushered in the new era of neutrino astronomy: the idea of using neutrinos, rather than light, to study the universe.
"Ten years ago, if I were giving a neutrino astronomy talk, I would have put neutrino astronomy in air quotes," said Naoko Kurahashi Neilson, a physicist at Drexel University and member of the IceCube collaboration. "Ten years ago, we hadn't even seen a neutrino from outside our solar system. Now I don't put air quotes because everybody agrees you can do astronomy with neutrinos."
Since then, the IceCube team has identified one more potential cosmic neutrino source – the galaxy Messier 77, a starburst galaxy with a supermassive black hole at its center.
IceCube can do fundamental physics
Two recent discoveries showed IceCube can help physicists understand the intrinsic properties and behaviors of neutrinos, even though it was not designed to do so. Neutrinos come in three "flavors," a particle physics term for the species of elementary particles: electron, muon and tau neutrinos. Researchers have so far identified two candidate tau neutrinos.
Physicists know neutrinos can change their flavor but not fully how or why this happens. IceCube's observation of the two tau neutrinos means cosmic neutrinos are changing flavor somewhere on their journey across the universe, a process predicted by physics but difficult to observe.
Additionally, researchers detected an electron antineutrino indicative of a Glashow resonance event. This is an extremely rare type of interaction between an electron antineutrino and an atomic electron – a type of particle interaction never observed before. Physicist Sheldon Glashow first theorized the interaction in 1960, but only IceCube's detection of an electron antineutrino in 2016 proved it happens in reality.
"It's incredible that we could actually achieve this," said Francis Halzen, a physicist at the University of Wisconsin-Madison and principal investigator of the IceCube collaboration said. "I'm a particle physicist, and this to me is just mind-blowing.
What's next for IceCube?
There are still many unanswered questions about cosmic neutrinos, but scientists suspect some will be answered in the next 10 years.
Halzen hopes IceCube can help physicists understand where cosmic rays – high-energy charged particles that transfer their energy to neutrinos – come from. Unlike neutrinos, cosmic rays are charged, so their paths through the universe are warped by magnetic fields, making it nearly impossible for physicists to know where they came from without other information.
Kurahashi Neilson hopes researchers can learn more about cosmic particle accelerators and when and how often they spew out neutrinos. "We're at the tip of an iceberg, right? And we don't know how big or deep or what shape the iceberg is. We know there are neutrino sources. We've maybe seen one or two, so what are the rest? When do they come out? How often? How are they distributed? What does the universe look like in neutrinos?" she said.