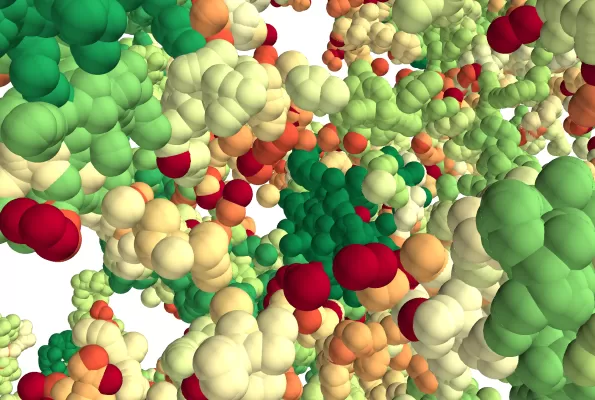
Beyond DNA: How scientists are learning to control gene function
NSF Emerging Frontiers in Research and Innovation program funds advancements in tissue regeneration, gene therapy, DNA mobility and epigenetic editing
Nearly every cell in your body contains the exact same DNA, from your skin cells to your brain cells. But how does a cell know how and when to turn into skin, muscle or brain?
Imagine that the DNA in cells is a long, twisted ladder made of billions of tiny building blocks. This DNA ladder carries all the instructions that tell your body how to grow, function and repair itself. When stretched out, the ladder in each human cell is 2 meters long, and it is difficult to imagine how it fits inside. Chromatin is how life solves this problem. Think of chromatin as a way of organizing DNA to fit within the nucleus (the control center of a cell). Chromatin is made of DNA wrapped around special proteins called histones to form a structure that looks like beads on a string, which is then looped and tightly compacted into chromosomes. This way DNA can be packed into a small space and unpacked whenever the cell needs access to genetic information.
DNA contains both coding and non-coding sequences. Proteins, which are essential cellular building blocks and mediators, are built using instructions contained in specific segments of coding DNA known as genes. Non-coding DNA plays a crucial supporting role by controlling when and how these genes are turned on or off for expression into proteins. Many non-coding regions enable chromatin interactions that regulate its structure and dynamics. For cells to become distinct tissues, many genes must be turned on and off across different DNA regions and over time. Chromatin organization can control this process — tightly packed chromatin restricts access to genes, keeping them off, whereas loosely packed chromatin allows genes to be turned on and expressed. Chromatin organization is influenced by chemical modifications of DNA and histone proteins, which thereby affect gene expression.
Thus, chromatin not only solves the problem of fitting DNA into a cell, it also provides a mechanism for regulating how the information in DNA is used.
Even though people inherit a fixed set of genes, their expression can be influenced by many factors throughout their lives, including environmental factors such as diet, stress and exposure to pollution. This phenomenon, called epigenetics, controls the identity and function of cells, in addition to the genetic sequence in DNA. To fully understand and potentially manipulate a cell's destiny, researchers must understand both its genetics and epigenetics.
EFMA and EFRI
Every two years, the Office of Emerging Frontiers and Multidisciplinary Activities (EFMA) in the Directorate for Engineering at the U.S. National Science Foundation identifies out-of-the-box research topics for the NSF Emerging Frontiers in Research and Innovation (EFRI) program. Under four-year grants, interdisciplinary teams work on transformative, high-risk, high-reward projects and to tackle the biggest challenges facing the nation.
In 2018 and 2019, EFRI focused on chromatin and epigenetic engineering to find new ways to control how genes are turned on and off. Through deeper knowledge and novel tools, researchers can engineer gene expression for many applications, including combatting disease, boosting crop plant performance or developing organisms that can remediate environmental damage.
Turning cancer cells off
Vadim Backman focuses on understanding and controlling chromatin organization. His team developed a high-resolution genome imaging platform to visualize chromatin in 3D, enabling more accurate predictions for genome engineering outcomes.
Backman’s interdisciplinary team combines genome biology with physics to model genome functions. They classify cellular features, like DNA structure and accessibility to predict the likelihood of gene activity from chromatin edits. This precise manipulation has applications in cancer treatment, organ regeneration, injury prevention, and reversing aging.
The team is developing drugs and interventions targeting cells affected by cancer or oxygen loss from strokes or heart attacks. For example, they developed an electromagnetic simulation technique that alters chromatin and gene expression, enabling heart cells to quickly repair damaged tissue.
Revealing how DNA gets rearranged inside the cell
Megan King's goal was to understand the relationship between chromatin structure and its functions and to engineer a device to measure changes in chromatin mobility.
King and her team discovered that a special protein complex called INO80 is an important driver of chromatin movements inside the nucleus and are engineering a device to watch chromatin interactions happening in real time inside living cells. Previous methods analyzed millions of cells in aggregate at a single time. The new device can look at what is happening in a single cell over many time points. This is crucial for understanding the complexity of tissues of many different cell types, like the brain or immune system.
Folding genetic information
Carlos Castro has made important advances with his team in delivering DNA into cells using nanostructures. Using principles from origami paper folding to create intricate designs, researchers can package genetic information very tightly within these nanostructures, enabling the delivery of even the longest genes into the nucleus. This new technology offers a safer, more cost-efficient alternative to traditional viral gene therapy, with potential applications in treating diseases and improving live cell imaging.
Additionally, DNA origami structures can control how gene products interact with cell components, enabling the manipulation of cell properties and functions. This capability could be used in tissue engineering to create artificial tissues and organs.
Epigenetic editing to control gene expression and combat disease
Charles Gersbach's project uses the cancer-associated gene called MYC as a case study to test how changes in chromatin architecture lead to changes in gene expression and tumor characteristics. The team developed new genome-editing technologies to specifically target the non-coding regulatory regions of DNA that turn genes on or off.
This approach can add or remove chemical modifications (epigenetic marks), mimicking changes that might occur in nature in response to the environment. This epigenome engineering approach, which addresses variations in the non-coding genome linked to disease susceptibilities, can improve disease interventions. An epigenetic editing company, Tune Therapeutics, was founded to develop new therapies based on this research.
Empowering Future Innovators
Many EFRI teams leverage the NSF Research Experience and Mentoring program to provide paid research experiences and mentoring to broaden participation and include more diverse talents in engineering. Backman's team offers an opportunity for high school students and undergraduates to participate in research. King supports undergraduates from underrepresented minority groups and/or first-generation, low-income college students to begin their careers. Castro enables undergraduates to experience research merged with technology development and entrepreneurship.
The EFRI projects have yielded groundbreaking advancements in the understanding and manipulation of gene expression. Supported by interdisciplinary research and mentoring programs, these collaborative efforts have advanced scientific knowledge and fostered a new generation of scientists equipped to tackle complex challenges in genetic and epigenetic engineering.