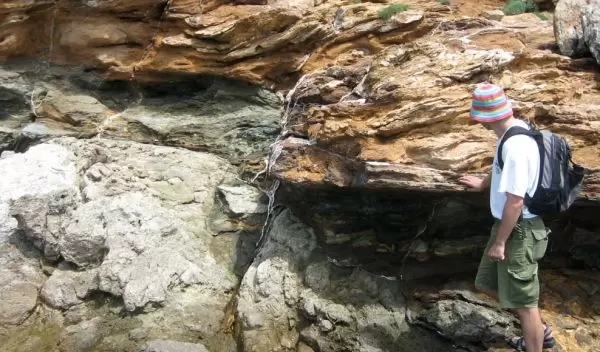
Mystery Behind Weak Earthquake Faults Solved
A chance meeting and a common interest in tectonic faults took Cristiano Collettini and Chris Marone to the Isle of Elba to sample a tectonic fault that breaks most of the rules of fault mechanics. Their work reveals why these faults slip.
In May 2008, Cristiano and I were at a workshop in the Italian Apennines to discuss a possible drilling project into the low-angle, normal faults (ones that occur when the Earth's crust is stretched) in that region. The faults in that region are an enigma because standard analysis shows that they shouldn't exist.
Cristiano had worked in this region and knew of a well-studied, low-angle, normal fault on the Isle of Elba, the Zuccale Fault. It is exposed on a beach on the far side of the main island, beyond the prison complex where Napoleon was exiled in 1814. We began talking about a trip to Elba during the meeting.
My family was on sabbatical in Rome during this time, and my wife had just had our fifth child, Massimo, in late March.
Cristiano's wife had also recently given birth to their second son, Simone. Neither of our children was yet a champion sleeper.
Because our sabbatical was over at the end of June, we didn't have much time left to collect the samples, but how could we justify the fieldwork trip to our families?
I couldn't leave my wife at home with all five kids (Massimo's older siblings are Tino, 2; Linda, 3; Dan, 14; and Vicki 17) and Cristiano had a similar problem (Simone's brother, Claudio is the same age as Tino, 2).
We solved the problem by bringing Vicki, Tino and Claudio to Elba with us.
We arrived on a late afternoon in mid-June. The walk from the beautiful beach to the fault was easy at first, but as we got closer, the beach narrowed and we began a rather rigorous climb (for 2-year-old legs) up to the terrace where the fault was exposed.
As soon as we got there, the boys wanted to run up to the edge of the 15-foot cliff bounding the terrace, but once they saw we were collecting rocks, they dug right in to "help." Soon the boys were bleeding from minor cuts and they began throwing rocks off the cliffs. That was our cue that it was time to retreat to the beach.
The next day, we enlisted Vicki to keep the boys at the beach. By mid-afternoon, we had collected a suite of samples, and the boys hadn't accumulated any more scrapes.
Cristiano and the rocks arrived at Penn State in October to begin the lab-friction experiments. We wanted to see if the fault rocks were weak enough to explain why low-angle, normal faults are active in that region. I had done similar studies on fault rocks before, so we started with the standard approach, which is to crush the rock samples and construct layers of rock powder that we could shear (abrade).
But Cristiano was insistent that we also measure the properties of the intact rock, by shearing it in the orientation it existed in within the fault zone. Andre Niemeijer, who was then a post-doc in my lab, and Igor Faoro, an Italian graduate student, had been working to develop methods for cutting fragile samples, so we started testing ideas about how we could create a sample that was roughly 5 centimeters (cm) by 5 cm by 1.0 cm and which had the fault zone fabric parallel to the main sample faces.
Luckily, we had quite a bit of sample, because the first several attempts failed. Then, Igor had a revelation and set up a makeshift sculpting studio by duct-taping a Shopvac hose to the leveling jig that held the sample and using a Dremel tool to sculpt the blocks.
When we began to run experiments, we noticed a problem right away. The rock powders had typical coefficients of friction (~ 0.6), but the solid wafers of rock--that Andre and Igor were by now experts at sculpting--produced much lower values. We were perplexed because we had made the powders and wafers from exactly the same fault rock samples, so the material properties should have been identical.
It's not uncommon to find experiment-to-experiment variability in friction due to heterogeneities in the rocks, but differences this large were unheard of. Even though all the samples were labeled in the field and packed in labeled containers, I thought we must have mixed them up. So, we made more powders and wafers and started again.
The result was the same, and now we were perplexed. The wafers were not perfectly homogeneous, but there was nothing visible to explain such large differences in steady-state sliding friction. After we reproduced this curious result three times, on different pieces from the same fault zone unit, I decided to take the wafers, after shearing, and powder them. That way we'd be sure that the bulk chemistry was the same in both cases.
We took each of the wafers and crushed them, and then made layers with the powders. To our surprise, the new powders had friction values of ~ 0.6! It wasn't until we made thin sections and started to think about the thin- (less than 10 millionths of a meter) but-very-abundant seams of clays that we realized these fabric elements in the rock were acting in concert to produce a form of lubrication.
The role of fabric in rock deformation at high temperature had been well known for many years, but as a community, geophysicists working on faults in the brittle field had not considered that they could be so important as a possible mechanism for fault weakening.
Read more about the work in the press release It's Not Your Fault.
-- Chris Marone , Penn State University, marone@psu.edu, and Cristiano Collettini, Universita degli Studi di Perugia, Italy, colle@unipg.it
This Behind the Scenes article was provided to LiveScience in partnership with the National Science Foundation.