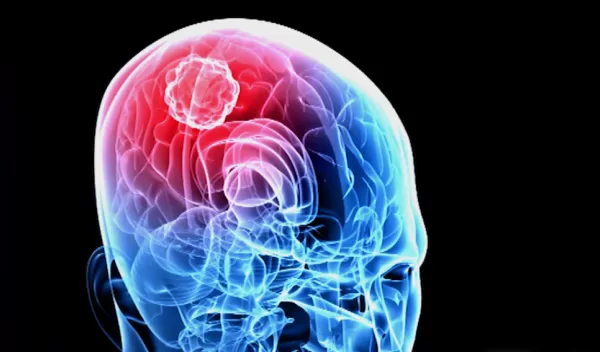
Radically modified cells may stunt brain tumor growth
For B-roll footage of this story please contact Cliff Braverman, cbraverm@nsf.gov.
The American Brain Tumor Association says this year nearly 70,000 people in the United States will be diagnosed with tumors that form in blood vessels, cranial nerves, lymphatic tissue and other parts of the brain. Of those, nearly 12,000 people will be diagnosed with a particularly deadly form of brain cancer called glioblastoma multiforme (GBM).
GBMs hide behind a protective barrier in the brain and, among other things, attack white blood cells that serve as the body's defense. Now, with some innovative science, National Science Foundation (NSF)-funded researchers are working to improve the ability of those same white blood cells to attack the cancer right back.
Stefan Bossmann and Deryl Troyer at Kansas State University in Manhattan, Kan., are developing a new materials treatment method that uses a type of white blood cell called a neutrophil to slip medications past the brain's protective barrier and strike down malignant tumors directly.
More than 100 years ago, when blue dye was injected into the bloodstream of an animal, scientists discovered that tissues in the animal's whole body turned blue, except in its brain and spinal cord. They determined that a "blood-brain barrier" protects the brain from extraneous materials in the blood. It was later determined that the blood-brain barrier also protects tumors in the brain from being readily exposed to anticancer drugs.
"Defensive cells--essentially, white blood cells--have the ability of moving through [the blood-brain barrier] to tumors and metastases," explains Bossmann. "The goal of our research is to use cells as transport ships for anticancer drugs."
In principle, using cells to carry drugs to intended targets is a pretty straightforward concept. However, creating a "cargo hold" within the cells that is sturdy enough to successfully carry a medicinal payload past the blood-brain barrier to a desired endpoint has been a challenge.
Previous efforts have resulted in cargo holds that leak, burst prematurely or fuse with the cells that carry them, causing the drugs to be released before reaching the target and killing the transport cells, not the tumors.
For doctors treating patients with GBM, new treatment methods are sorely needed.
"Innovation in designing new therapeutic strategies is urgently needed to tackle GBM, one of the most lethal human tumors and the most aggressive adult brain tumor," says Erwin Van Meir, a brain tumor researcher and director of the Winship Cancer Institute's Cancer Cell Biology Program at Emory University in Atlanta, Ga.
"The challenge has been that tumors have developed strategies to avoid immune detection, or they render the immune cells [helpless] or even switch them to a state that helps tumor growth," he says. "Developing cell-based therapeutics in new ways is certainly exciting."
A new class of "cages"
To solve the problem of getting drugs past the blood-brain barrier and directly to tumors, Bossmann and Troyer are developing a new class of protease-activatable polymer-caged liposomes (PPCLs). Liposomes essentially are artificial bubbles created within cells that can be used as vessels to carry and administer therapeutic medicines.
The researchers are creating self-assembling "cages" that wrap around liposomes--turning them into more secure cargo-holds. Their process involves loading caged liposomes with anticancer medicine before up-take by neutrophils that will self-destruct and release the drugs when they reach tumors.
The PPCLs proposed by the researchers are designed to be more stable than classic liposomes, prevent systemic leaking during transport and activate only once they integrate into tumors. This should facilitate the killing of fast growing tumor cells and slow growing cancer stem cells responsible for the reappearance of tumors and the formation of metastases that spread tumors to other parts of the body.
The proposed cell therapy method would work by taking whole blood from cancer patients, then loading redesigned cargo holds within the whole blood's neutrophils with anticancer drugs and afterwards re-injecting the modified neutrophils into the patient's blood stream.
"The advantage of using defensive white blood cells is that they are patient-derived. This means that they are available in sufficient numbers for repetitive treatments," explains Troyer, an expert in veterinary medicine and preclinical models of cancer at Kansas State. "It also means there are absolutely no issues of rejection of the transport cell by the patient's immune system."
Chemo, nano, cell
Currently physicians use two basic methods to deliver anticancer drugs: traditional chemotherapy and nanotherapy.
Chemotherapy delivers drugs via the infusion of liquid medicines directly into a vein, typically through an IV bag or drip chamber. The process also may involve delivering drugs by an injection into a layer of skin or orally as pills and capsules. Unfortunately, the process comes with potentially harmful side effects, including toxicity that can compromise liver, kidney and immune function. Chemo also may cause secondary malignancies or cancerous growths.
Meanwhile, nanotherapy uses nano-sized drug particles, on the order of about a billionth of a meter, to enhance drug-dosage performance. Particles at this size move more easily through the blood-brain barrier and can saturate tumors at higher rates, providing faster therapeutic action. Still, they deliver only about 10 percent of a therapeutic dose of anticancer drugs--better than the 1-2 percent delivered by chemotherapy, but still inefficient.
"Neither classic chemotherapy nor conventional nanotherapy is capable of delivering more than a fraction of a prescribed dosage of anticancer drugs to tumors and metastases," says Bossmann, a professor of chemistry at Kansas State. "Consequently, other cells of the body are damaged, including stem cells in bone marrow that form immune cells, as well as stem cells in the digestive system. Cell therapy offers the opportunity to transport more than 50 percent of anticancer drugs to their target, while leaving the patient's immune system intact."
"If they can actually do that and deliver the amount of drugs that they think they can, it could make a difference," says Mark Dewhirst, director of Duke University's Tumor Microcirculation Laboratory in Durham, N.C., "a big difference." Dewhirst, who has published more than 400 peer-reviewed articles, book chapters and reviews, is one of a number of interested observers.
A new standard of care
The project, "Neutrophil Delivery of Apoptosis-Inducing Anticancer Drugs," is one of 40 projects funded in the first round of an NSF initiative that addresses extremely complicated and pressing scientific problems. Called INSPIRE, the initiative funds potentially transformative research that does not fit neatly into any one, scientific field, but crosses disciplinary boundaries.
"New ideas oftentimes come when researchers from disciplines other than the traditional ones attack a frustrating problem as they see it from a novel perspective and have different methodologies they can put to use," says Van Meir. "Cancer has been the realm of oncological and biological disciplines for decades and we need others to join in our efforts to solve the problem."
"More than half of the patients with GBM will die within a year, and more than 90 percent within three years," says the Director of NSF's Biophotonics program Leon Esterowitz. "The results from this project will exploit patient-specific, tumor-homing cells for treatment delivery and could lead to a new standard of care for brain cancers."
NSF's Biomaterials program in its Directorate for Mathematical and Physical Sciences (MPS) funds the research. It is also co-funded by NSF's Biophotonics program along with its Materials Surface Engineering program, both in the Directorate for Engineering.
"The focus of this INSPIRE project is to develop basic scientific knowledge of the materials that are being studied," says Joseph Akkara, director of the Biomaterials program in MPS. "In a larger sense, biomedical applications are at present supported by the National Institutes of Health."
Uphill challenges
Bossmann and Troyer already have successfully synthesized PPCL prototypes and used 4T1 breast cancer--a well-known mouse model for metastasizing cancer--to prove that tumors are capable of attracting large numbers of defensive white blood cells.
Still, these preliminary results face uphill challenges.
Pathology examinations, for example, reveal that neutrophils are rarely seen in human brain cancers, even though the cancers produce signaling molecules that attract neutrophils. In fact, research has shown another type of defensive cell, the macrophage, plays a more prominent role than neutrophils in the growth of brain cancers.
"So, the challenge is to figure out how to enable neutrophil trafficking into glioblastoma," says Dewhirst. "Using the cells by themselves, without some local stimulus to attract them may not work."
Van Meir, who has authored more than 140 scientific publications on brain tumor biology and genetics, posits the findings from the researchers' preliminary breast cancer model may not be directly applicable to brain cancers as a result.
Bossmann and Troyer are aware of the issue, and now in the second year of the research grant, their team is developing protocols to attract neutrophils to brain tumors in mice.
Creating a condition that causes tumors to attract white blood cells is only one of the remaining obstacles, however. Determining the optimum size of the PPCLs for effective drug delivery; reducing impediments to the health of delivery cells during transport; determining what to expect from white blood cells loaded with medicines that can become toxic when they do not reach the tumor and designing protocols that stimulate, not deactivate, the patient's immune system during cell therapy are some of the remaining concerns.
Still, "I am confident that our research will reach our goals," says Bossmann.
If successful, the strategy could expand to targeting other cell types. The researchers believe the method's principles could evolve into targeted therapies for viral, bacterial and protozoal infections. However, they acknowledge there is still a ways to go to truly beat cancer.
"Early recognition of cancer is vital, because early cancer is much easier to treat, compared to late stage cancer," says Troyer. "In addition, preventing or treating metastases and eradicating cancer stem cell populations by combined chemotherapy and immunotherapy are important keys to survival."
"Brain tumors remain a disease for which there are many challenges because of the eloquence of the site where they are," says Henry Friedman, an internationally recognized neuro-oncologist and deputy director of Duke's Preston Robert Tisch Brain Tumor Center. "No one therapy is going to be the magic bullet, but the more different interventions we have, the more likely we're going to be successful."
This new treatment method "is not going to be the only intervention necessary, but it certainly is going to be part of the spectrum of different therapies that we use," he says. "It is going to be one of additional weapons that may find a place in the treatment of malignant brain tumors."