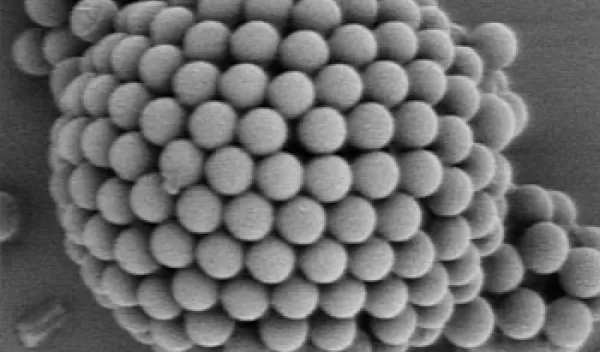
Researchers Solve 100-Year-Old Puzzle of How Layer of Particles Coats the Surface of a Sphere
In a discovery likely to impact fields as diverse as medicine and nanomanufacturing, researchers have determined how nature arranges charged particles in a thin layer around a sphere. The leap forward in understanding this theoretical problem may help reveal chinks in the armor of viruses and bacteria (revealing potential drug targets) and guide engineers designing new molecules.
On a flat surface, particles that repel each other will arrange themselves to create a stable energy state, eventually settling at vertices within a lattice of identical triangles much like billiard balls at the start of a game.
Yet, for nearly a century, researchers studying spherical structures have known that a flat lattice cannot be simply wrapped around a sphere because the lattice of perfect triangles breaks down. Since as early as 1904, when Nobel prize-winning physicist J.J. Thomson theorized about electron shells in atoms, researchers have wondered what structure the thin web of particles would choose if wrapped around a sphere.
In 2003, NSF-supported scientists Mark Bowick of Syracuse University, David Nelson of Harvard University and Alex Travesset of Iowa State University and Ames National Laboratory made a major breakthrough in the puzzle, supported by experiments with water droplets and tiny, self-assembling beads. The researchers described in the journal Science how spherical crystals compensate for the curved surface on which they exist by developing "scars" -- defects that allow the beads to pack into place.
"The theoretical work from our laboratories, and others, suggested that crystals on a curved surface would pack unusually, in a way not found in flat crystals," Bowick said, although the packing depends upon the size of the crystal relative to the surface particles.
The researchers were joined by experimentalists Andreas Bausch and Michael Nikolaides of Technische Universität München in Germany and Angelo Cacciuto of the FOM Institute for Atomic and Molecular Physics in the Netherlands, along with NSF-supported researcher David Weitz and his research team at Harvard University. With experimentation, the team was able to test, and ultimately support, their models of how spherical crystals form in various natural settings.
"This study's interplay between theory and experiment reveals fascinating insights," said Daryl Hess, the NSF program officer who oversees support for the project. "This is curiosity driven research, [but] these findings will likely have impact across many fields of science."
Unlike previous approaches using computer models to determine how the charged particles arrange themselves, the new research involves experimentation, creating and observing the defects in the crystal structure and determining how the particles find the most stable arrangement.
To create the spherical crystals, the researchers coaxed polystyrene beads only one micron in diameter to congregate around tiny balls of water tens of microns in diameter suspended in an oily mixture.
The team then used a light microscope to view the spheres and digitally traced images of the crystalline patterns. Whereas a flat crystal pattern would consist of a regular pattern of adjacent, equilateral triangles, the researchers found the triangular pattern of the spherical crystal was disrupted and squeezed due to defects (a bead would have five or seven close neighbors instead of the six it would in a perfect lattice).
"We found that curvature can fundamentally change the arrangement of particles on the surface," Bowick said.
Smaller spheres had 12 isolated defects, but larger spheres showed jagged strings of defects the researchers dubbed scars. The scars are a coalescence of simple defects in the lattice pattern that begin and end within the crystal, unlike similar structures in flat crystals which begin and end at crystal surfaces. The researchers observed that the scars erupt in a predictable way based upon the size of the sphere and consistent with the predictions of their theory.
"These structures are a signature of the curved geometry and do not depend on the details of the particle interactions on the surface," Bowick said. "The scars should appear in any type of spherical packing or crystallization."
In addition to confirmation of the researchers' approach, the new findings also shed light on how such structures form and persist in nature. Some viruses, such as the monkey cancer virus SV40, and some bacteria have similar spherical structures. Knowledge of scar formation may reveal how to target chemical reactions at those sites, potentially leading to treatments for similar pathogens.
The research also offers clues about some of the most prevalent structures in nanoscale science and engineering, the fullerenes. Knowing how defects can arise within nanostructures may help researchers devise better methods to create fullerenes or other large molecules with desirable characteristics.
-- Josh Chamot