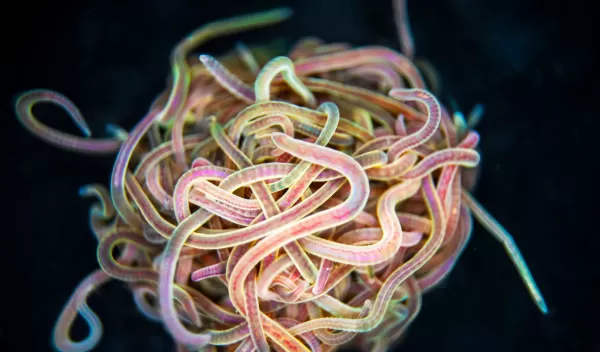
Unraveling the mathematics behind wiggly worm knots
For millennia, humans have used knots for all kinds of reasons — to tie rope, braid hair or weave fabrics. But there are organisms that are better at tying knots and far superior — and faster — at untangling them.
Tiny California blackworms intricately tangle themselves by the thousands to form ball-shaped blobs that allow them to execute a wide range of biological functions. But, most striking of all, while the worms tangle over a period of several minutes, they can untangle in mere milliseconds, escaping at the first sign of a threat from a predator.
Saad Bhamla wanted to understand precisely how the blackworms execute their tangling and untangling movements. To investigate, Bhamla and a team of researchers at Georgia Tech linked up with mathematicians at MIT. Their research, published in Science, could influence the design of fiberlike, shape-shifting robotics that self-assemble and move in ways that are fast and reversible. The study also highlights how cross-disciplinary collaboration can answer some of the most perplexing questions in disparate fields.
Fascinated by the science of ultrafast movement and collective behavior, Bhamla and Harry Tuazon have studied California blackworms for years, observing how they use collective movement to form blobs and then disperse.
Tuazon, a co-first author of the study, collected videos of his experiments with the worms, including macro videos of the worms' collective dispersal mechanism and microscopic videos of one, two, three and several worms that capture their movements.
Bhamla and Tuazon approached mathematicians Jörn Dunkel of MIT and Vishal Patil of Stanford University about a collaboration. After seeing Tuazon’s videos, the two theorists, who specialize in knots and topology, were eager to join.
Bhamla and Tuazon set about finding an imaging technique that would allow them to peer inside the worm blob so they could gather more data. After much trial and error, they landed on an unexpected solution: ultrasound. By placing a live worm blob in nontoxic jelly and using a commercial ultrasound machine, they were finally able to observe the inside of the intricate worm tangles.
After analyzing the ultrasound videos, Tuazon and other researchers in Bhamla's lab painstakingly tracked the movement of the worms by hand, plotting more than 46,000 data points for Patil and Dunkel to use to understand the mathematics behind the movements.
"This observations' implications are far-reaching, as active filaments are ubiquitous in biological structures from DNA strands to entire organisms," said Eva Kanso, a program director in the U.S. National Science Foundation's Directorate for Engineering, which supported the research.
"These filaments serve myriad functions and can provide a general motif for engineering multifunctional structures and materials that change properties on demand. Just as the worm blobs perform remarkable tangling and untangling feats, so may future bioinspired materials defy the limits of conventional structures by exploiting the interplay between mechanics, geometry and activity."