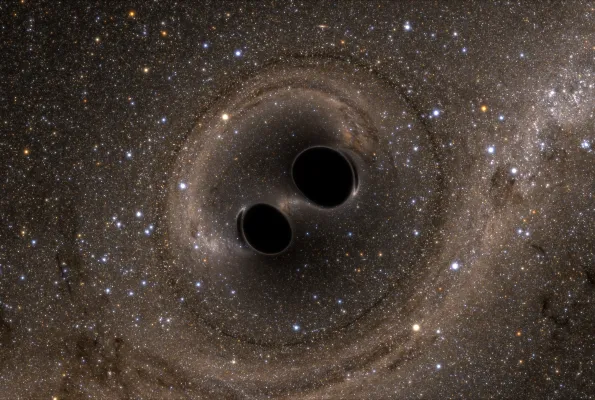
A black hole collision: From theory to evidence
The creation of a black hole is one of the most violent events in the universe. Formed from the explosive collapse of a massive star, a black hole is a monster that consumes everything that comes too close, including light. The matter around some black holes are sources of huge amounts of electromagnetic radiation -- the radiation enables us to “see” the black holes. And when a black hole collides with another black hole, space itself moves.
The gravitational waves that result from colliding black holes were first predicted by Albert Einstein in 1916. Unlike a regular wave of light or even an ocean wave of water, a gravitational wave is not carried by any particles. Instead, a gravitational wave occurs when the fabric of space-time itself waves or vibrates. This tiny phenomenon would obviously be very difficult to observe directly, but a massive event such as a black hole collision could theoretically produce such a detectable result – if you had a sensitive enough way to measure it.
Enter LIGO, the Laser Interferometer Gravitational-Wave Observatory. Read on to learn more about the NSF-funded facility that finally provided direct confirmation of the existence of gravitational waves and has given us a new way to look at what happens when black holes collide.
1970s and 1980s
NSF began funding research on the development of gravitational wave detectors in the late 1970s, using a technique called interferometry. An interferometer, like LIGO for example, works by detecting interference between two sources of light. If two light waves have the same wavelength, they’ll overlap and create a pattern of dark and bright spots. LIGO operates on this principle, with light sent down two long (4 km, or 2 ½ miles!) arms and reflected back to the central detector by mirrors at the ends of the arms. Any fine movement of the mirrors – or, more precisely, the space around them, would cause a difference in the returning light from at least one arm. If one arm is stretched slightly, the interference pattern at the detector will change.
1990s and 2000s
LIGO is actually two sister facilities, one located in Hanford, Washington, and the other in Livingston, Louisiana. With two observatories so far apart, scientists could be more confident that something detected by both facilities was a real signal, and not local vibrations in the Earth. When the decade-long construction process for the pair of facilities was completed in 1999, observations could finally begin.
Since LIGO has been in operation, it has supported researchers and students working on complex astrophysical questions. And while LIGO didn’t find evidence of gravitational waves with its original design, it was still helping to answer important questions. Early findings helped theorists “rule out” certain models meant to describe neutron stars and even the Big Bang, refining our understanding of the universe. LIGO was setting itself up to be an incredibly sensitive device, able to detect the tiniest of tiny distance changes between its arms (shorter than one ten-thousandth the width of a proton!) and able to reliably distinguish between vibrational noise and real signals.
LIGO also became a resource for teachers and families in surrounding communities. The Science Education Center at Livingston opened in 2006, and construction began on a new Exploration Center at Hanford last year.
2010s and today
Taking lessons from its first decade of operations, LIGO greatly revamped its design, and plans for Advanced LIGO were born. These advanced upgrades were completed in 2015, and LIGO promptly broke the big scientific news it was designed for: Gravitational waves had been found!
The gravitational wave signal, an audio chirp, deftly proved Einstein’s nearly 100-year-old prediction and opened the door to a new age of multi-messenger astrophysical research. With a reliable way to detect gravitational waves, researchers now have a new signature to look for in space. In the six years since this initial discovery, LIGO has identified dozens of black hole merger events. It has also observed a handful of other gravitational wave events caused by the collision of neutron stars -- the dense, dead stars remaining after supernova explosions.
In the past year alone, LIGO has produced a number of stunning discoveries: the merger of two unequally sized black holes, the detection of a mysterious object that could be the heaviest neutron star or the lightest black hole ever discovered, the finding of the largest gravitational wave source yet detected, and evidence that teeny quantum fluctuations can indeed be enough to move their sensitive mirrors.
Just two short years after the initial discovery of the gravitational waves, LIGO’s founders, Barry Barish and Kip Thorne of Caltech and Rainer Weiss of MIT, were awarded the 2017 Nobel Prize in physics for their multi-decade, pioneering work on LIGO. And just this year, on February 3, the Institute for Electrical and Electronics Engineers awarded LIGO and its Italian counterpart facility, Virgo, their Milestone award for that same breakthrough discovery.
NSF funded the documentary crew that was there to capture the historic chirp. You can watch the award-winning documentary on the groundbreaking LIGO discoveries, which is streaming for free on Vimeo. The documentary includes the Nobel Prize ceremony.
The journey into black hole and gravitational wave research isn’t over yet, so stay tuned for future exciting developments.